TAU study discovers how foreign DNA can evade and neutralize bacterial defense systems
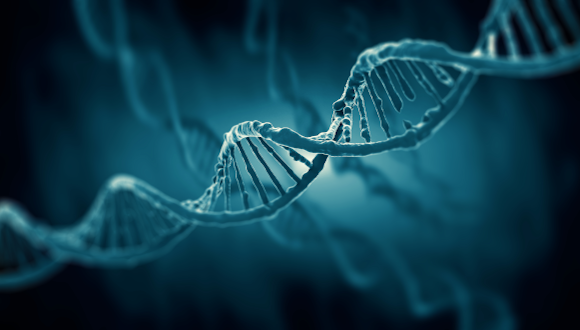
Findings could lead to development of methods to combat antibiotic resistance
Support this researchA new study from Tel Aviv University (TAU) researchers reveals how bacterial defense mechanisms can be neutralized, enabling the efficient transfer of genetic material between bacteria.
The researchers believe this discovery could pave the way for developing tools to address the antibiotic resistance crisis and promote more effective genetic manipulation methods for medical, industrial, and environmental purposes.
The study was led by PhD student Bruria Samuel from the laboratory of Professor David Burstein at the Shmunis School of Biomedicine and Cancer Research at TAU’s George S. Wise Faculty of Life Sciences. The findings were published on October 9, 2024, in the journal Nature.
Genetic diversity is essential for the survival and adaptation of different species in response to environmental changes. For humans and many other organisms, sexual reproduction is the primary driver of the genetic diversity required for survival. However, bacteria and other microorganisms lack such a reproduction mechanism. Nevertheless, as demonstrated by the alarming speed at which antibiotic resistance spreads among bacterial populations, bacteria have alternative mechanisms to maintain the genetic diversity necessary for survival, including the direct transfer of DNA between bacteria.
DNA transfer between bacteria plays a crucial role in their survival. But a key aspect of this process has remained underexplored: how is the exchange of genetic material so prevalent despite bacteria having a wide range of defense mechanisms designed to destroy any foreign genetic material entering their cells?
The new research focuses on a process called “conjugation,” one of the main mechanisms for transferring DNA from one bacterium to another. During conjugation, one bacterial cell connects directly to another through a tiny tube that allows the transfer of genetic material fragments known as plasmids.
“Plasmids are small, circular, double-stranded DNA molecules classified as ‘mobile genetic elements,’” Professor Burstein explains. “Like viruses, plasmids move from one cell to another, but unlike viruses, they do not need to kill the host bacterium to complete the transfer.”
As part of the natural exchange, plasmids often provide recipient bacteria with genetic advantages. For example, many antibiotic-resistance genes spread through plasmid transfer between bacteria. However, bacteria also have numerous defense mechanisms aimed at eliminating any foreign DNA entering their cells.
“Conjugation is a well-known process that scientists also use in the lab to transfer genes between bacteria. It’s also known that bacteria possess mechanisms to destroy foreign DNA, including plasmid DNA, and some of these mechanisms are even used for various research purposes. However, until now, no one has fully explored how plasmids overcome these defense mechanisms,” says Professor Burstein.
Samuel conducted a computational analysis of 33,000 plasmids and identified genes associated with “anti-defense” systems that help plasmids bypass bacterial defense mechanisms. In order to pass through the thin tube that connects the bacteria, one of those circular strands is cut at a certain point by a protein, which then binds to the cleaved strand and initiates its transfer to the recipient cell.
“The genes for the anti-defense systems that I identified were found to be concentrated near that cutting point, and organized in such a manner that they would be the first genes to enter the new cell,” Samuel says. “This strategic positioning allows the genes to be activated immediately upon transfer, giving the plasmid the advantage needed to neutralize the recipient bacteria’s defense systems.”
The next step was to demonstrate in the lab that this phenomenon indeed occurs during plasmid transfer between bacteria. “We used plasmids that confer antibiotic resistance and introduced them into bacteria equipped with CRISPR, the well-known bacterial defense system that can target and destroy DNA, including that of plasmids,” Samuel explains. “This method allowed us to easily test the conditions under which the plasmid could overcome the defense system — if it succeeds in overcoming the CRISPR system, the recipient bacteria become resistant to antibiotics. If it fails, the bacteria die.”
Using this method, Samuel demonstrated that if the anti-defense genes are positioned near the DNA entry point, the plasmid successfully overcomes the CRISPR system. However, if these genes are located elsewhere on the plasmid, the CRISPR system destroys the plasmid, and the bacteria die upon exposure to antibiotics.
Professor Burstein notes that understanding the positioning of anti-defense systems on plasmids could enable the identification of new anti-defense genes, a subject currently under highly active research. “Another potential application could involve designing effective plasmids for genetic manipulation of natural bacterial populations,” he says. “This could help block antibiotic resistance genes in hospital bacterial populations, teach bacteria in soil and water to break down pollutants or fix carbon dioxide, and even manipulate gut bacteria to improve human health.”
Ramot, TAU’s technology transfer company, regards this discovery as a significant biotechnological breakthrough with broad applications. “First, I want to congratulate Professor Burstein and his lab team on this fascinating scientific discovery,” Dr. Ronen Kreizman, CEO of Ramot, says. “The new research opens revolutionary possibilities in areas such as developing drugs against resistant bacteria, synthetic biology, agritech, and foodtech. The ability to control and fine-tune genetic material transfer between bacteria could become a powerful tool for addressing environmental, agricultural, and medical challenges. We are currently working on commercializing this technology to realize its full potential.”
Other contributors to the research include Dr. Karin Mittelman, Shirly Croitoru, and Maya Ben-Haim from Professor Burstein’s lab.